Ultimately, this knowledge could enable engineers to boost the efficiency of chemical plants, power plants and oil refineries, or any place where hot particles and cold fluid mix.
The find contributes to the fundamental understanding of a decades-old problem, explained L.S. Fan, a Distinguished University Professor and the John C. Easton Professor of Engineering in the Department of Chemical and Biomolecular Engineering at Ohio State.
Fan described his findings at the national meeting of the American Chemical Society on March 28, as he received the E.V. Murphree Award in Industrial and Engineering Chemistry.
In the 1950s, scientists who recreated industrial chemical conditions in the lab noticed something interesting about the way two objects collide. When a flat solid surface is much hotter than a liquid droplet, the droplet never makes direct contact with the solid surface. The droplet dances around on the hot surface without touching it.
Fan likened the effect to what happens when water hits the surface of a hot cooking pan.
"The water sizzles and dances around," he said. "It's the same for droplets in contact with spherical particles in a chemical reactor. But then the question becomes, is the heat effectively transferred during the contact? And it turns out that only a very small amount of the heat is transferred due to a very short contact time."
Though scientists knew of this phenomenon for decades, they were not exactly sure how it happened. The technology needed to answer the question - specifically, the numerical simulation required to produce highly detailed three-dimensional characteristics of the process - were not attempted until recently.
Fan and his team derived equations to explain how heat travels between separated surfaces. Then they compared three-dimensional supercomputer simulations based on their equations to experiments on actual collisions in the laboratory.
They found that, as heat flows from the surface of a hot particle to a cold droplet, a vapor layer forms between them. The vapor layer forms a cushion that buffers the droplet's impact, so that it bounces off the particle. Heat is exchanged during that brief contact, but the particle and droplet never actually touch, because the vapor layer forms a high-pressure zone that the droplets cannot overcome.
"Once that vapor layer forms, it would take infinite force to bring the droplet and the particle together," Fan said.
In the computer simulations, the high-pressure zone sometimes pushed back against the heated droplet so that it bounced away, or it broke up when it rebounded.
When Fan's team compared the simulations to experiments in the lab, the behavior of the droplets matched almost exactly.
Scientists can apply this finding in industries such as oil refining, where hot-and-cold objects routinely interact. For example, the Ohio State researchers are now testing how different mixes of particles and droplets affect heat flow to help the droplets evaporate.
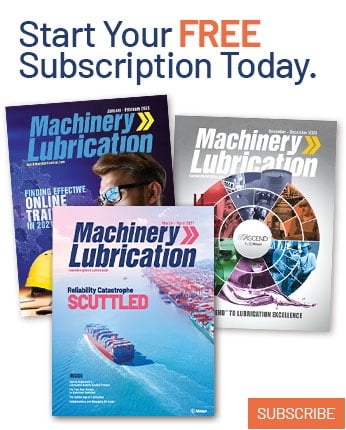